Color and Light
Here we ask how color is sensed by the viewer. To answer the question we need to specify how color is described and how color information is received by the eye. The starting point of an understanding of color is a description of light.
Light: Photons and Waves
Isaac Newton discovered in 1672 that light could be split into many colors by a prism, and used this experimental concept to analyze light. The colors produced by light passing through a prism are arranged in a precise array or spectrum from red through orange, yellow, green, blue, indigo and into violet. The students' memory trick is to recall the name "Roy G. Biv" where each letter represents a color. The order of colors is constant, and each color has a unique signature identifying its location in the spectrum. The signature of color is the wavelength of light.
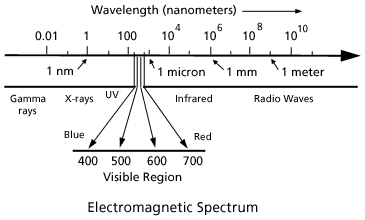
Fig. 1. The electromagnetic spectrum, which encompasses the visible region of light, extends from gamma rays with wave lengths of one hundredth of a nanometer to radio waves with wave lengths of one meter or greater.
Somewhat less than 100 years after Newton's discoveries, James Clerk Maxwell showed that light was a form of electromagnetic radiation. This radiation contains radio waves, visible light and X-rays. Figure 1 shows electromagnetic radiation as a spectrum of radiation extending beyond the visible radiation to include at one end radio waves and at the other end gamma rays. The visible light region occupies a very small portion of the electromagnetic spectrum. The light emitted by the sun falls within the visible region and extends beyond the red (into the infrared) and the ultraviolet (UV) with a maximum intensity in the yellow.
When we consider light as an electromagnetic wave, a color's spectral signature may be identified by noting its wavelength. We sense the waves as color, violet being the shortest wavelength and red the longest. Visible light is the range of wavelengths within the electromagnetic spectrum that the eye responds to. Although radiation of longer or shorter wavelengths are present, the human eye is not capable of responding to it.
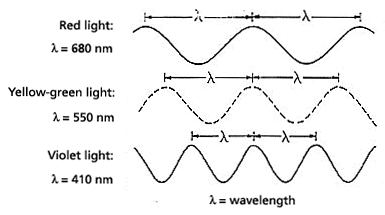
Figure 2. A wave representation of three different light hues: red, yellow-green and violet, each with a different wavelength λ, which represents the distance between wave crests.
Three typical waves of visible light are shown in Fig. 2. The wavelength is the distance from one wave crest to the next, and is represented by the Greek letter lambda, l. Violet light is electromagnetic radiation with wavelengths of 410 nanometers and red light has a wavelength of 680 nanometers.
The nanometer is a unit of distance in the metric scale and is abbreviated as nm. One nanometer (nm) equals one thousand millionths of a meter (m) or 1 nm = 10-9 m. One nanometer is a distance too small to be resolved in an optical microscope but one micron (µm) or one thousand nanometers can be resolved (1 micron = 1000 nm). The wavelengths of visible light are smaller than common objects such as the thickness of a sheet of paper or the diameter of a human hair. Both of these are about one hundred microns thick which translates to distances greater than one hundred wavelengths of visible light.
As we move through the visible spectrum of violet, blue, green, yellow, orange and red, the wavelengths become longer. The range of wavelengths (400 - 700 nm) of visible light is centrally located in the electromagnetic spectrum (Fig. 1). Infrared and radio waves are at the long wavelength side while ultraviolet (UV), x-rays and gamma rays lie at the short wavelength side of the electromagnetic spectrum. Radiation with wavelengths shorter than 400 nm cannot be sensed by the eye. Light with wavelength longer than 700 nanometers is also invisible.
We can describe light as electromagnetic waves with color identified by its wavelength. We can also consider light as a stream of minute packets of energy-photons - which create a pulsating electromagnetic disturbance. A single photon of one color differs from a photon of another color only by its energy.
In the description of light, the most convenient unit of energy to use is the electron volt, abbreviated eV. The electron volt is the energy gained by an electron that moves across a positive voltage of one volt (V). For example 1.5 electron volts is the energy gained by an electron moving from a negative metal plate to a positive plate which are connected to the terminals of a common 1.5 volt "C" batter.
Visible light is composed of photons in the energy range of around 2 to 3 eV (Fig. 3). As the energy of the light increases, the wavelength decreases. Orange light with a wavelength of 620 nanometers is composed of photons with energy of 2 eV. It is the energy range of 1.8 to 3.1 eV which triggers the photo receptors in the eye. Lower energies (longer wavelengths) are not detected by the human eye but can be detected by special infrared sensors. Higher energies (shorter wavelengths) such as x-rays are detected by x-ray sensitive photographic film or again by special devices.
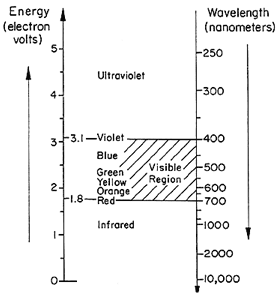
Figure 3. Diagram showing the visible region of the electromagnetic spectrum in terms of wavelength and corresponding energies. The visible region extends from 400 nm to 700 nm (wavelength) with corresponding energies of 3.1 to 1.8 electron volts (eV).
Light rays are composed of photons whose energy specifies a color from red to violet. The intensity or brightness of the light is defined by the flux, or number of photons passing through a unit area in a unit time; i.e., number of photons per cm2 per sec.
If we specify a wavelength in the visible range on the electromagnetic scale, we can attribute a color to it. That is, laser light with a single wavelength of 650 nanometers looks red. We show the major spectral colors in Fig. 4a as a linear sequence from red (at 700mm) to violet at 400 nm. A circular sequence of these same spectral colors, the color wheel first attributed to Isaac Newton, is shown in Fig. 4b. The progression of colors from red through violet is identical to that on the linear scale. The circular wavelength scale outside the color wheel shows the wavelength connection between the linear and circular sequences. The purple region in the color wheel is a notable difference between the two sequences. Colors in this purple portion of the color wheel are composed of mixtures of wavelength and cannot be represented by a single wavelength.
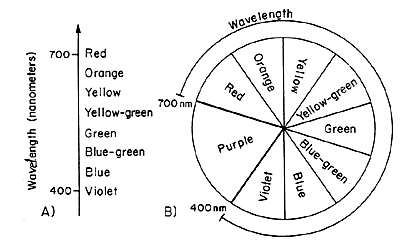
Figure 4. The region of visible light in wavelengths shown as a linear arrangement (a) and as a circle (b) as conceived by Sir Isaac Newton. The color purple shown in the color wheel (b) is composed of a mixture of light in the red and violet regions of the spectrum. Purple cannot be represented by a single wavelength of light.
No single wavelength exists for the color brown just as none exists for purple. Purple can be created with a mixture of wavelengths in both the red and the violet. Brown requires a more complex mixture of wavelengths from at least three regions of the sequence.
The Color of Objects
Here we consider the color of an object illuminated by white light. Color is produced by the absorption of selected wavelengths of light by an object. Objects can be thought of as absorbing all colors except the colors of their appearance which are reflected as illustrated in Fig. 5. A blue object illuminated by white light absorbs most of the wavelengths except those corresponding to blue light. These blue wavelengths are reflected by the object.
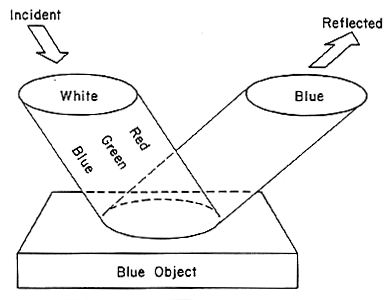
Figure 5. White light composed of all wavelengths of visible light incident on a pure blue object. Only blue light is reflected from the surface.
The Eye and Color Sensation
Our perception of color arises from the composition of light - the energy spectrum of photons - which enter the eye. The retina on the inner surface of the back of the eye (Fig. 6) contains photosensitive cells. These cells contain pigments which absorb visible light. Of the two classes of photosensitive cells, rods and cones, it is the cones that allow us to distinguish between different colors. The rods are effective in dim light and sense differences in light intensity - the flux of incident photons - not photon energy. So in dim light we perceive colored objects as shades of grey, not shades of color.
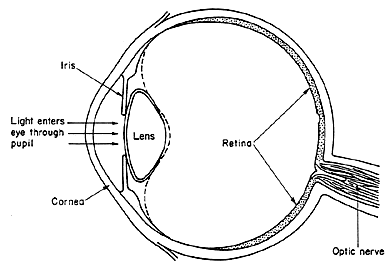
Figure 6. A cross-sectional representation of the eye showing light entering through the pupil. The photosensitive cells, cones and rods, are located in the retina: cones respond to color and rods respond to light intensity.
Color is perceived in the retina by three sets of cones which are photoreceptors with sensitivity to photons whose energy broadly overlaps the blue, green and red portions of the spectrum. Color vision is possible because the sets of cones differ from each other in their sensitivity to photon energy. The sensitivity of the cones to light of the same intensity (the same photon flux) but different wavelengths (energy) is shown in Fig. 7. The maximum sensitivity is to yellow light, but cone R has a maximum in the red-orange, G in the green-yellow, and B in the blue. The sensitivities of the three cones overlap. For every color signal or flux of photons reaching the eye, some ratio of response within the three types of cones is triggered. It is this ratio that permits the perception of a particular color.
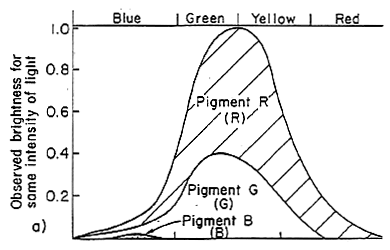
Figure 7. The response of the three cones to incident light: cone R (pigment R) has a maximum sensitivity in the orange-red, cone G (pigment G) in the green-yellow, and cone B (pigment B) in the blue portions of the visible spectrum. The sensitivities of the three cones overlap and the perceived color is due to the relative response of the three cones.
Want to try activities highlighting these principles? Click on the names of these activities to find easy, hands-on experiments: